Osmosis Meaning
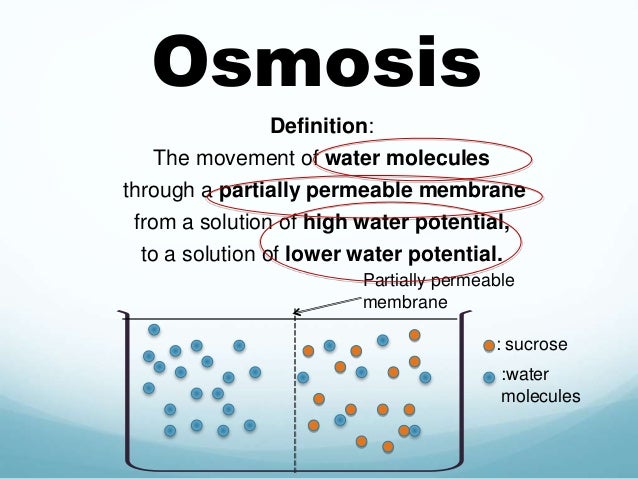
Marta Zaccaria. Claudio Ronco, in, 2019 OsmosisOsmosis is a biophysical phenomenon occurring commonly in biologic systems, in which cells of fluid compartments are separated by semipermeable membranes. Osmosis describes the diffusion of the solvent through a semipermeable membrane. The driving force of the solvent shift is the concentration difference of solutes in the solutions separated by the semipermeable membrane. In contrast to solvent, solutes cannot pass this barrier. Water, the usual solvent in biologic systems, migrates from the compartment with lower concentration to the compartment with higher concentration of solutes. The net fluid flux ends when the concentration of osmotic active molecules is equal in the two compartments.
Osmosis definition: Osmosis is gradual absorption. (noun) An example of osmosis is slowly learning how to sew after sitting next to an experience seamstress. Osmosis is the process where solvent molecules move through a semipermeable membrane from a dilute solution into a more concentrated solution (which becomes more dilute). In most cases, the solvent is water. However, the solvent may be another liquid or even a gas. Osmosis can be made to do work.
Therefore the distribution of water is a matter of osmosis and not transport of solutes. Osmosis is a special type of diffusion, namely the diffusion of water across a semipermeable membrane. Water readily crosses a membrane down its potential gradient from high to low potential ( Fig. 19.3) 4.
Osmotic pressure is the force required to prevent water movement across the semipermeable membrane. Net water movement continues until its potential reaches zero. An early application of the basic principles of osmosis came from the pioneering work on hemolysis of red blood cells by William Hewson in the 1770s (see Chapter 2). It has also been discussed that MLVs (multilamellar vesicles, liposomes) behave as almost perfect osmometers, swelling in hypotonic solutions and shrinking in hypertonic solutions (see Chapter 3) 5,6. Liposome swelling and shrinking can be easily followed by changes in absorbance due to light scattering using a simple spectrophotometer. Therefore, osmosis has been investigated for many years using common and inexpensive methodologies and a lot is known about the process. Osmosis and osmotic pressure.
Water is placed in a U-shaped tube where each of the tube arms is separated by a semipermeable membrane with pores of a size that water can easily pass through but a solute cannot. Upon addition of the solute to the tube's right arm, water diffuses from left to right (high water potential to low). The column of water in the tube's right arm (the one containing the solute) rises until the extra weight of the column equals the osmotic pressure caused by the solute. A pump could then be used to counter the osmotic pressure whereupon the solution columns in the right and left arms of the tube are made the same. The pump pressure required to equalize the height of the two columns is the osmotic pressure 4. Note a small amount of the solute leaks from right to left since no filter is perfect.Membranes are rarely, if ever, perfectly semipermeable. Deviation from ideality is defined by a reflection coefficient ( σ).
For an ideal semipermeable membrane where a solute is totally impermeable, σ = 1. If a solute is totally permeable (its permeability is equal to water), σ = 0. Biological membranes are excellent semipermeable barriers with σ = 0.75 to 1.0. Baumgarten, Joseph J. Feher, in, 2012 IIIA. Definition of OsmosisOsmosis refers to the movement of fluid across a membrane in response to differing concentrations of solutes on the two sides of the membrane. Osmosis has been used since antiquity to preserve foods by dehydration with salt or sugar.
The removal of water from a tissue by salt was referred to as imbibition. This description comes from the notion that these solutes attracted water from material they touched. In 1748, J.A. Nollet used an animal bladder to separate chambers containing water and wine. He noted that the volume in the wine chamber increased and, if this chamber was closed, a pressure developed. He named the phenomenon osmosis from the Greek ωσμoς, meaning thrust or impulse.
First strike: final hour apk. Let your kid to choose a lovely character to watch them fall asleep while listening to a calming tune.Games for kids.
Pfeffer (1877) provided early quantitative observations on osmosis. He made an artificial membrane in the walls of an unglazed porcelain vessel by reacting copper salts with potassium ferrocyanide to form a copper ferrocyanide precipitation membrane on the surface of the vessel.
I think this is the answer!! I can`t wait to talk to my hubby about taking the bottom out of the crib!! We have been waiting with baited breath for her toddler sleep sack to arrive in the mail, which it did this morning, but I fear it will only make a small dent, if any.
He used this membrane to separate a sucrose solution inside the vessel from water outside and found a volume flow from the water side to the sucrose side. Pfeffer observed that the flow was proportional to the sucrose concentration.
Further, a pressure applied inside the vessel produced a filtration flow proportional to the pressure. He found that a closed vessel containing a sucrose solution would develop a pressure proportional to the concentration of sucrose. He recognized this as an equilibrium state in which the pressure balanced the osmosis caused by the sucrose solution. Pfeffer’s original data for the osmotic pressure of sucrose solutions are plotted in Fig. He defined osmotic pressure as the hydrostatic pressure necessary to stop osmotic flow across a barrier (e.g.
A membrane) that is impermeable to the solute. This concept is illustrated in Fig. Osmotic pressure is a property intrinsic to the solution and is measured at equilibrium, when the pressure-driven flow exactly balances the osmotic-driven flow. By defining osmotic pressure in this way, we assign a positive value to an apparent reduction in pressure brought about by dissolving the solute.
Thus, fluid movement occurs from the solution of low osmotic pressure (water) to the solution of high osmotic pressure, opposite in direction to the hydraulic flow of water from high to low hydrostatic pressure. Plot of data from Pfeffer (1877) for the osmotic pressure of sucrose solutions. A copper ferrocyanide precipitation membrane was formed in the walls of an unglazed porcelain cup.
The membrane separated a sucrose solution in the inner chamber from water in the outer chamber. The inner chamber was then attached to a manometer and sealed. The linear relation between the pressure measured with this device and the sucrose concentration were the experimental impetus for deriving van’t Hoff’s law. Equivalence of hydrostatic and osmotic pressures in driving fluid flow across a membrane. (A) An ideal, semipermeable membrane is freely permeable to water, but is impermeable to solute.
When the membrane separates pure water on the right from solution on the left, water moves to the solution side. This water flow is osmosis. The flow, Q v, in cm 3s −1, is linearly related to the difference in osmotic pressure, Δπ, by the area of the membrane, A, and the hydraulic conductivity, L p. Positive Q v is taken as flow to the right. The flow causes expansion of the left compartment and movement of the piston (which is assumed to be weightless). (B) Application of a pressure, P L, to the left compartment forces water out of this compartment, across the semipermeable membrane. The flow is linearly related to the pressure difference between the two compartments.
(C) Application of a P L so that Δ P = Δπ results in no net flow across the membrane. The osmotic pressure of a solution is defined as the pressure necessary to stop water movement when the ideal, semipermeable membrane separates water from the solution.An ideal semipermeable membrane is required for determining osmotic pressure. These membranes are permeable to water but absolutely impermeable to solute. The concept of osmotic pressure differs from tonicity in that tonicity compares two solutions separated by a specific non-ideal membrane. If the membrane is highly permeable to solute as well as to water, no water flow will occur and, therefore, the externally applied pressure required to stop osmosis is zero.
This observation makes it plain that the effective osmotic pressure, which is measured with a real membrane, must be due to some interaction of the membrane with the solute because pressure depends on both the specific solute and the specific membrane. Osmosis refers to the movement of fluid across a membrane in response to different concentrations of solutes on the two sides of the membrane.
The movement of fluid is toward the more concentrated solution. Osmotic pressure is defined as the pressure that must be applied to the solution side to stop fluid movement when a semipermeable membrane separates a solution from pure water. Here, the semipermeable membrane is permeable to water but not to solute. The osmotic pressure for dilute ideal solutions obeys van’t Hoff’s Law. Q v = A L p ( Δ P − σ Δ π )Osmosis and osmotic pressure is a thermodynamic concept which exists independently of mechanism.
In microporous membranes, osmosis is caused by a momentum deficit within the pores due to the reflection of solute molecules by the membrane. This reduces the pressure on the solution side of the pore by π for a semipermeable membrane.Thus there are three characteristic parameters for describing passive material transfer across membranes: the permeability, p, the hydraulic conductivity, L p, and the reflection coefficient, σ.Osmolarity is a kind of concentration measure, distinct from molarity. It is related to other colligative properties of solutions including freezing point depression, vapor pressure depression, and boiling point elevation. Tonicity is a related concept but involves a real, biological membrane that may not be semipermeable.
Tonicity makes reference to a particular cell and its membrane. Solutions may be isoosmotic but not isotonic. Osmosis, the natural movement of water into a solution through a semipermeable membrane, has been employed in the development of zero-order release drug delivery systems ( Fig.
Only water, not solutes, can diffuse through the semipermeable membrane ( Herbig et al., 1995). For different polymeric membranes, their water vapor transmission value is distinguishable, and the selection of a semipermeable membrane depends on the nature of application. The rate of release of drug from these products is determined by the constant flow of water across a semipermeable membrane into a reservoir that contains an osmotic agent called as osmogen. The rate of release is constant and can be controlled within fixed limits, yielding relatively constant drug concentration.
The osmotic pump is similar to a reservoir device but it contains an osmogen. Osmogen is the active agent in salt form, which acts to imbibe water from the surrounding medium through a semipermeable membrane ( Gupta et al., 2011). Pressure is developed within the reservoir which forces the active agent out of the reservoir device through an orifice. The rate of release can be adjusted by changing the osmogen and size of the orifice. The constant release is unaffected by the environment of the gastrointestinal tract and relying simply on the passage of water into the dosage form, acts as an advantage for this type of system ( Lachman et al., 1991).
Consequently, osmotically controlled systems can be classified in to single chamber osmotic pump (elementary osmotic pump) and multichamber osmotic pump (push-pull osmotic pump, osmotic pump with nonexpanding chamber) ( Fig. Specific types: are controlled porosity osmotic pump, monolithic bursting osmotic pump, osmotic bursting osmotic pump, osmotic release oral system-colon targeted (OROS-CT), multiparticulate delayed release systems, and liquid oral osmotic system ( Vyas and Khar, 2000; Bechgaard and Nielson, 1978).
Monteiro, in, 2017 DefinitionsOsmosis: This is the hydrostatic force acting to equalize the concentration of water on both sides of the membrane that is impermeable to substances dissolved in that water. Water will move along its concentration gradient. This force is termed osmotic pressure or, in the case of colloids, e.g., albumin, oncotic pressure.
It is proportional to the number of atoms/ions/molecules in solution and is expressed as mOsm/liter (osmolarity) or mOsm/kg (osmolality) of solution. The cell membrane and the capillary membrane are both partially permeable membranes, although not strictly semipermeable in the chemical sense. They act, however, as partial barriers dividing the ECF from the ICF space, and the intravascular from the interstitial space. Osmotic or oncotic shifts occur across these membranes, modified by physiological as well as pathological mechanisms.Osmolarity describes the molar number of osmotically active particles per liter of solution. In practice, the osmolarity of a solution can be “calculated” by adding up the milliequivalent concentrations of the various ions in the solution.
Box 49.2Calculation of the Osmolarity of 0.9% SalineThe molecular weight of NaCl is 58.430.9% NaCl contains 9 g NaCl/1000 mL solutionMolarity of 0.9% solution = 9 g/L/58.43 g/mol = 0.154 mol/L or a 154 mmol/L solution of NaClSince each molecule of NaCl dissociates into Na + and Cl − ionsMolar value is multiplied by 2 = 308 = osmolarity of 0.9% saline.Colloid osmotic pressure: Osmolarity/osmolality is determined by the total number of dissolved “particles” in a solution, regardless of their size. Colloid osmotic pressure is the osmotic pressure generated by large molecules (e.g., albumin, hetastarch, dextran). Box 49.3Calculation of Osmotic PressureOsmotic pressure π = CRTC = 0.001 mmol/LR = 0.08206T = 273K+36K = 309K(body temperature)Hence π = CRT = (0.001)(0.08206)(309°)° = 0.02535 atm or 19.27 mmHgHemodilution: The beneficial effects of hemodilution are based on the correlation of hematocrit and whole blood viscosity. As the hematocrit and viscosity decrease, the cerebrovascular resistance correspondingly decreases and cerebral blood flow increases. However, one argument against hemodilution is that the oxygen carrying capacity of the blood also decreases. However, experimental evidence suggests that a hematocrit of 33% provides an optimal balance between viscosity and oxygen carrying capacity, and this has been applied clinically. Holden, in, 2015 1.1.3 Membrane proteinsBeyond motion via osmosis, DIB networks have no function without incorporated membrane proteins.
So far, there are two general strategies for incorporating membrane proteins into DIBs, namely, reconstitution or in situ synthesis. In reconstitution, membrane proteins are first purified from some form of biological synthesis, such as expression in bacteria or cell-free expression. On the other hand, proteins may be produced directly in the droplet by replacing the aqueous solution with a cellular expression extract and a DNA plasmid containing the gene of interest ( Syeda, Holden, Hwang, & Bayley, 2008). Each method has unique advantages and disadvantages. For example, purified proteins are easier to work with and can be diluted to known concentrations with relatively controlled activity. Also, reconstitution essentially guarantees that only one type of protein will be incorporated into the DIB. However, very few membrane proteins are robust enough to be purified from an expression system intact.
Indeed, this process has been restricted to mostly prokaryotic small channels and toxins so far. It can also be difficult to control the orientation of incorporated species using reconstitution techniques.
While some protein toxins like α-hemolysin (αHL) incorporate unidirectionally, others, like OmpG can insert with the intracellular or extracellular domain facing the membrane leaflet where the proteins were added ( Hwang et al., 2008). Expression of proteins within the droplet offers the unique advantage of bypassing the tedium associated with protein expression and purification in bacterial systems. The expression of any protein is as simple as including the desired gene/genes into the expression extract. However, the rate of synthesis and incorporation is relatively uncontrolled and there is a substantial lag time between droplet formation and protein expression.
Also, synthesis extracts tend to have a detrimental effect on DIB stability. Our experience with extracts from Escherichia coli and HeLa cells demonstrates that DIBs tend to coalesce within 30 min ( Syeda et al., 2008). The instability of DIBs in the presence of extracts is a significant hurdle for the continued development of DIB techniques, though some interesting solutions are on the horizon. Though in-droplet protein expression has been shown to work for several proteins, it has yet to be used in droplet networks primarily because of stability issues. Therefore, the examples discussed herein exclusively use protein reconstitution as the source of membrane protein.
The majority of examples use engineered forms of the αHL toxin from Staphylococcus aureus. Ozer, Peter C. Brazy, in, 2009 A Movement of Water between Intracellular Fluid and Extracellular Fluid Regulated by Osmotic PressureThe principle of osmosis states that when a semipermeable membrane separates two fluid spaces, water will flow from an area of lower solute concentration to one of higher solute concentration to achieve equilibrium so that the osmotic pressures are balanced. In biologic systems, the cell membranes allow water to diffuse across them, but they are relatively impermeable to charged solutes (e.g., sodium, potassium) and large organic molecules (e.g., glucose).
Cell membranes have special transport systems (proteins or channels) that regulate the movement of these ions and molecules across the cell membrane. Small, uncharged molecules (e.g., urea, ammonia) diffuse across cell membranes quickly. The concentration of solutes in the compartment (molecules or ions dissolved in the water) determines the osmolality of the fluid, and this is expressed as mOsm/kg of water. In the steady-state condition, body water is distributed between ICF and ECF compartments so that the osmolality is the same in each compartment. Therefore, the plasma osmolality is a fairly accurate estimate of the total body osmolality. Plasma osmolality 2 (Sodium) (Glucose in mg / dl) / 18 (Blood urea nitrogen in mg / dl) / 2.8The sodium concentration is doubled because each sodium ion is balanced, usually with a monovalent anion (e.g., chloride, bicarbonate).
Although glucose and urea contribute to plasma osmolality, their contribution to the calculation is small compared with that of the plasma sodium concentration. Exceptions to this statement are the patient with hyperglycemia from uncontrolled diabetes mellitus and the patient with uremia (an extremely high blood urea nitrogen level). Her total body solute content would be 280 mOsm / kg water × 5 L (1 L of water weighs 1 kg) 9800 mOsm. Her intracellular fluid solute content is 280 mOsm / kg water × 23.5 L 6580 mOsm.
Her extracellular fluid solute content is 280 mOsm / kg water × 11.5 L 3220 mOsm.Her total body solute content would be 280 mOsm/kg water × 35 L (1 L of water weighs 1 kg) = 9800 mOsm.Her intracellular fluid solute content is 280 mOsm/kg water × 23.5 L = 6580 mOsm.Her extracellular fluid solute content is 280 mOsm/kg water × 11.5 L = 3220 mOsm. The solutes in each compartment generate the osmotic pressure. This pressure is created only by solutes that do not readily cross the membrane that defines the compartment. Solutes that freely cross the membrane (e.g., urea) cannot generate a gradient or osmotic pressure. When the solute concentration on one side of the membrane is different from the solute concentration on the other side and when the solutes cannot diffuse across the membrane, then an osmotic pressure gradient is established. Water will move quickly from the side of the lower osmotic concentration toward the side with the higher osmotic concentration until the osmotic gradient is abolished.
Thus, the volume of a compartment reflects the percentage of osmotic solutes in that compartment and the total amount of water available ( Fig. In Section IV, the ways in which changes in compartment size occur will be discussed.
Diagrammatic representation of osmosis. When two compartments separated by a semipermeable membrane (dark tube) have unequal concentrations, only water can flow through the membrane to balance the concentrations of impermeant dissolved molecules. With this flow of water comes a subsequent change in compartment volume; thus, the two compartments that were once equal in size (solid line) have now shrunk or expanded on the basis of the presence of a lower or higher concentration, respectively (dashed line). Carney, in, 2010 12.12.3.4 Hyper- and HypoosmolalityThe principle of osmosis is central to all of biology, yet the potential osmotic load associated with administration of high doses of small molecules is rarely considered in toxicology. In addition to the direct hyperosmotic effects of chemical exposure ( Glasser et al. 1973), hyperosmolality can also result indirectly from dehydration, increased salt intake, loss of body water through vomiting, or inhibition of water reabsorption in the renal collecting duct (e.g., diabetes insipidus).
Conversely, compounds such as morphine, nicotine, certain tranquilizers, and anesthetics increase water reabsorption in the kidney and thus have the potential to induce hypoosmolality.As with acid–base balance, there are many maternal systems that tightly regulate extracellular fluid osmolality, among them the thirst centers in the brain that affect water intake, and arginine vasopressin that controls reabsorption of water in the renal collecting ducts. At the cellular level, sodium and chloride ion concentrations are the major determinants of osmotic pressure. Proteins in plasma and interstitial fluid are also an important source of osmotic pressure, usually referred to as oncotic or colloid osmotic pressure. This is particularly important in the movement of solutes across capillary walls.Studies on preimplantation embryos have demonstrated increased expression of genes such as p38 MAPK and CCM2 in response to hyperosmotic environments ( Fong et al. 2007), although we know that embryos at these early stages can be very sensitive to changes in osmolality. Limited data are available concerning osmoregulation during organogenesis, although one study ( Carney et al. 1996) showed that GD 10.5 rat conceptuses cultured with intact visceral yolk sac placenta grew normally in culture media that was extremely hypertonic (418 mOsmol kg −1 H 2O, vs 299 for control media).
The visceral yolk sac placenta may play a crucial role in the maintenance of embryonic osmolality. For example, trypan blue treatment of embryos in vivo and in vitro caused lysosomal damage to the visceral yolk sac endoderm, a decrease in exocoelomic fluid osmolality, and a corresponding increase in the incidence of embryonic edema ( Rogers et al. The opposite effect was observed following treatment with leupeptin, an inhibitor of yolk sac lysosomal proteinase activity, which caused an increase in exocoelomic fluid osmolality and malformations characterized by decreased neural tube volume ( Daston et al.
Embryonic edema, blistering, and hemorrhage are common responses to many teratogenic agents, suggesting that the embryo may have a limited ability to cope with changes in osmolality.During early fetal stages, injection of hypotonic or hypertonic solutions into the mother brings about equivalent changes in maternal and fetal plasma osmolality ( Bruns et al. 1964; Dancis et al. The consequences of these changes are unknown, although there is some evidence from studies in pregnant sheep suggesting that prolonged fetal hyperosmolality (induced by maternal water deprivation) may imprint the hypothalamic–pituitary arginine vasopressin regulatory system ( Ramirez et al. Once the fetal kidneys become functional, osmotic challenges can be dealt with by concentration or dilution of the fetal urine and excretion of this urine into the amniotic cavity.
'the tendency of fluids to pass through porous partitions and mix with each other; the diffusion of fluids through membranes,' 1867, Latinized from osmose (1854), a shortened form of endosmose 'inward passage of a fluid through a porous septum' (1829), from French endo- 'inward' + Greek ōsmos 'a thrusting, a pushing,' from stem of ōthein 'to push, to thrust,' from PIE *wedhe- 'to push, strike' (source also of Sanskrit vadhati 'pushes, strikes, destroys,' Avestan vadaya- 'to repulse'). Figurative sense is from 1900. Related: Osmotic (1854, from earlier endosmotic); osmotically.
Others Are Reading
Dictionary Entries near osmosis
Oslo
Osmanli
osmatic
osmium
Osmond
osmosis
osprey
osseous
Ossetian
Ossianic
ossicle